Blog
- All Blogs
- Back
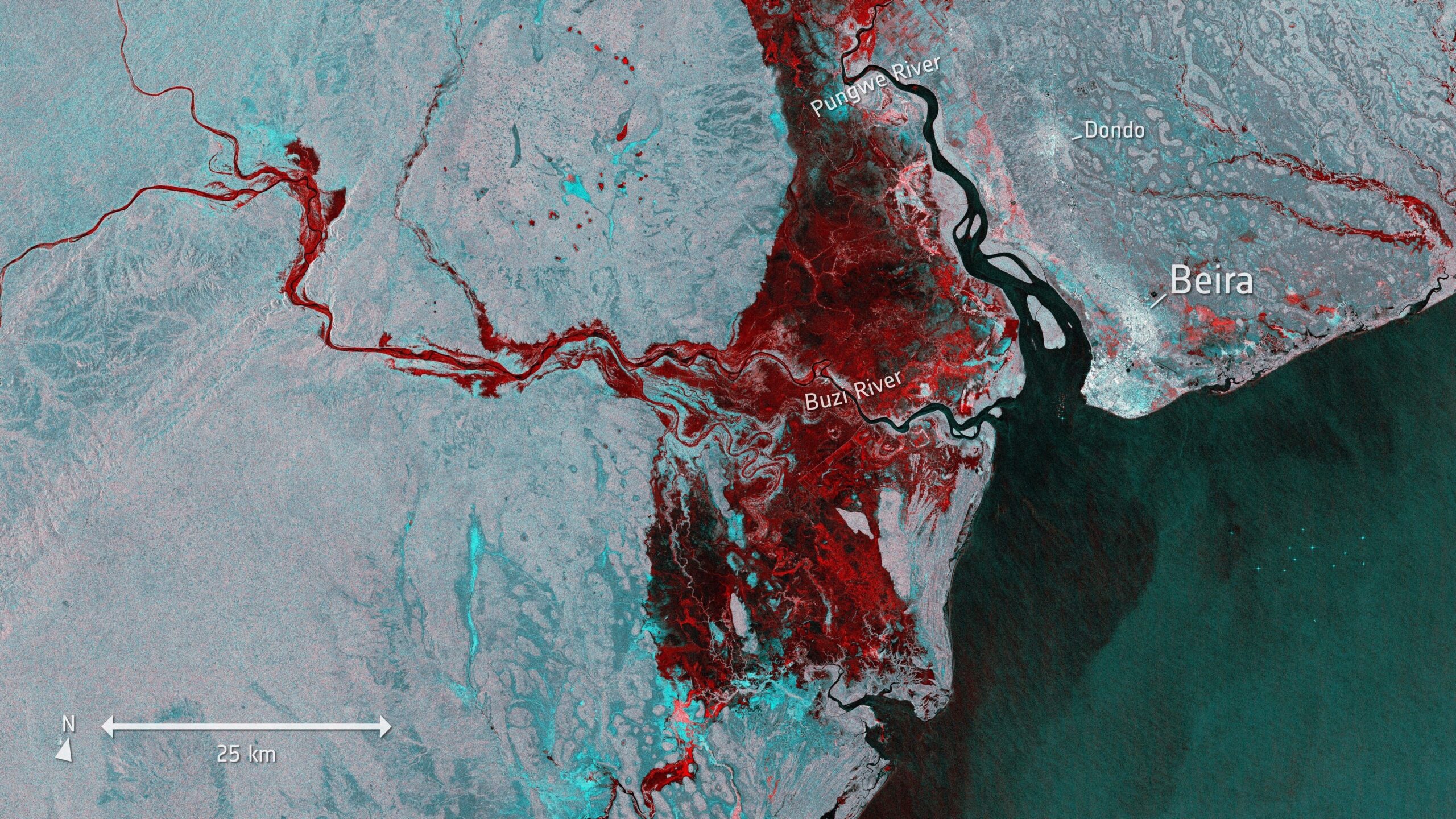
Synthetic Aperture Radar SAR is an active remote sensing technology that utilizes microwave signals to acquire detailed and precise images of the Earth’s surface and unlike…
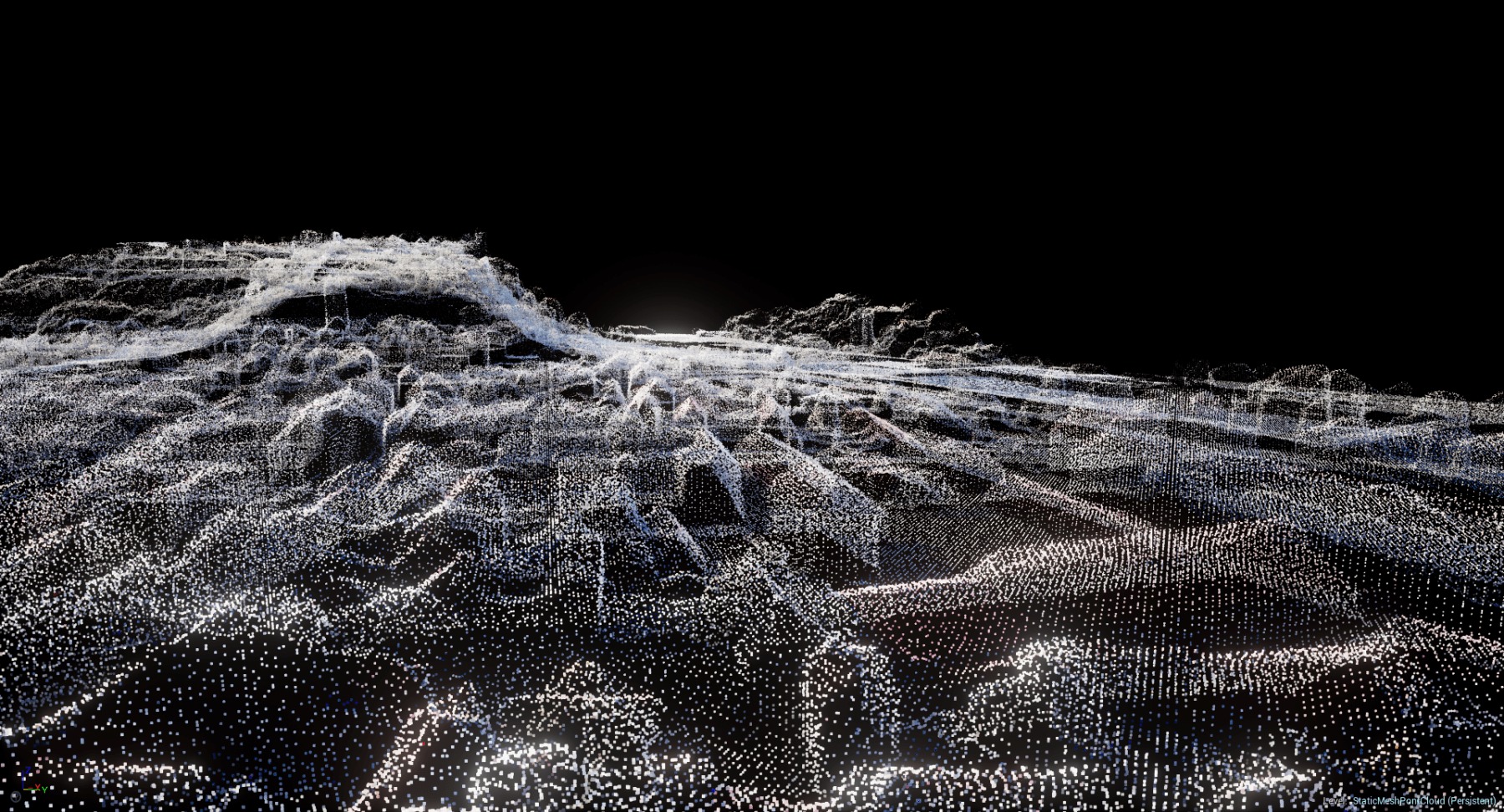
A collection of data points specified in three dimensions is called a point cloud. Concerning the physical world, every point in the cloud is uniquely identified…
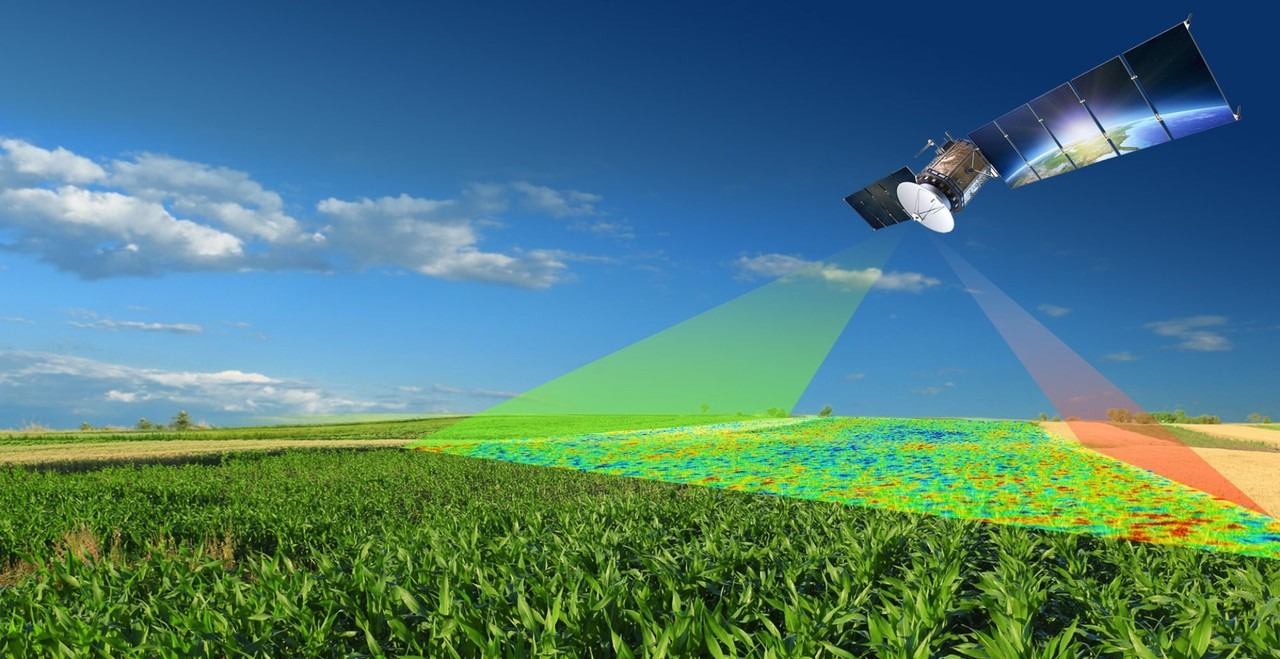
Technology has become a key factor in today’s agricultural scene guiding the sector toward previously unheard-of levels of sustainability and efficiency and one such pair of…
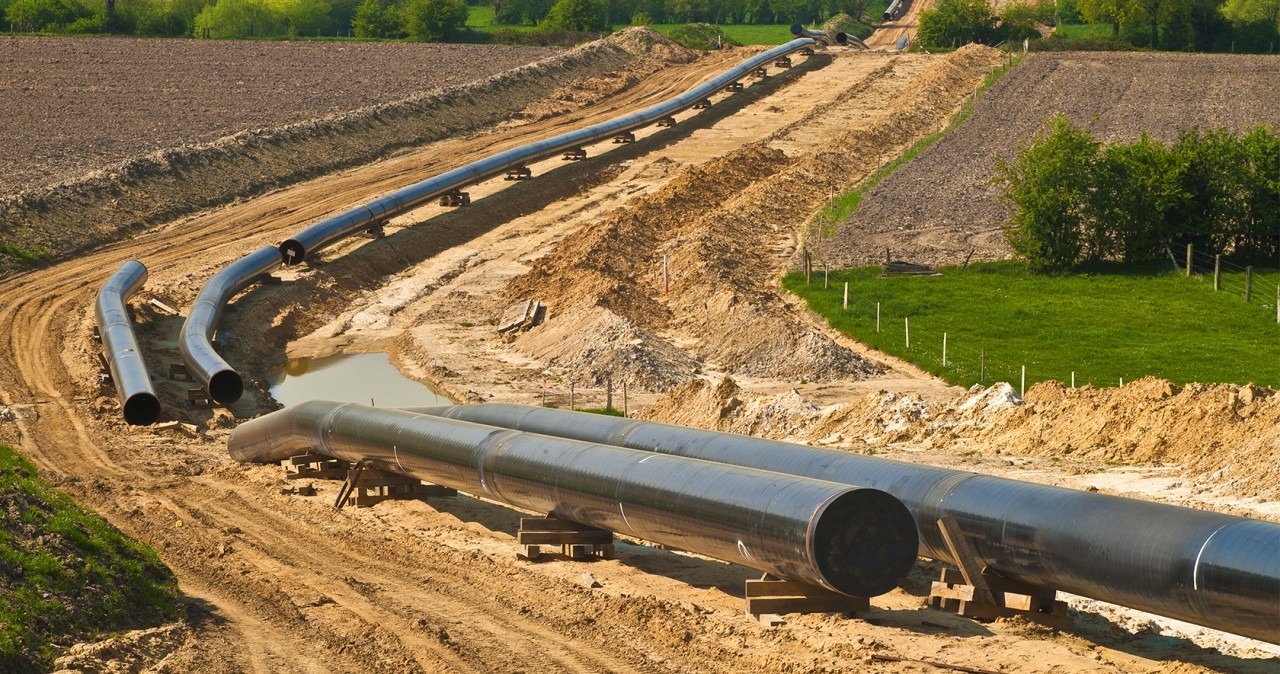
The efficient and secure transportation of oil and gas through pipelines is crucial and as the network of pipelines continues to expand globally, the need for…
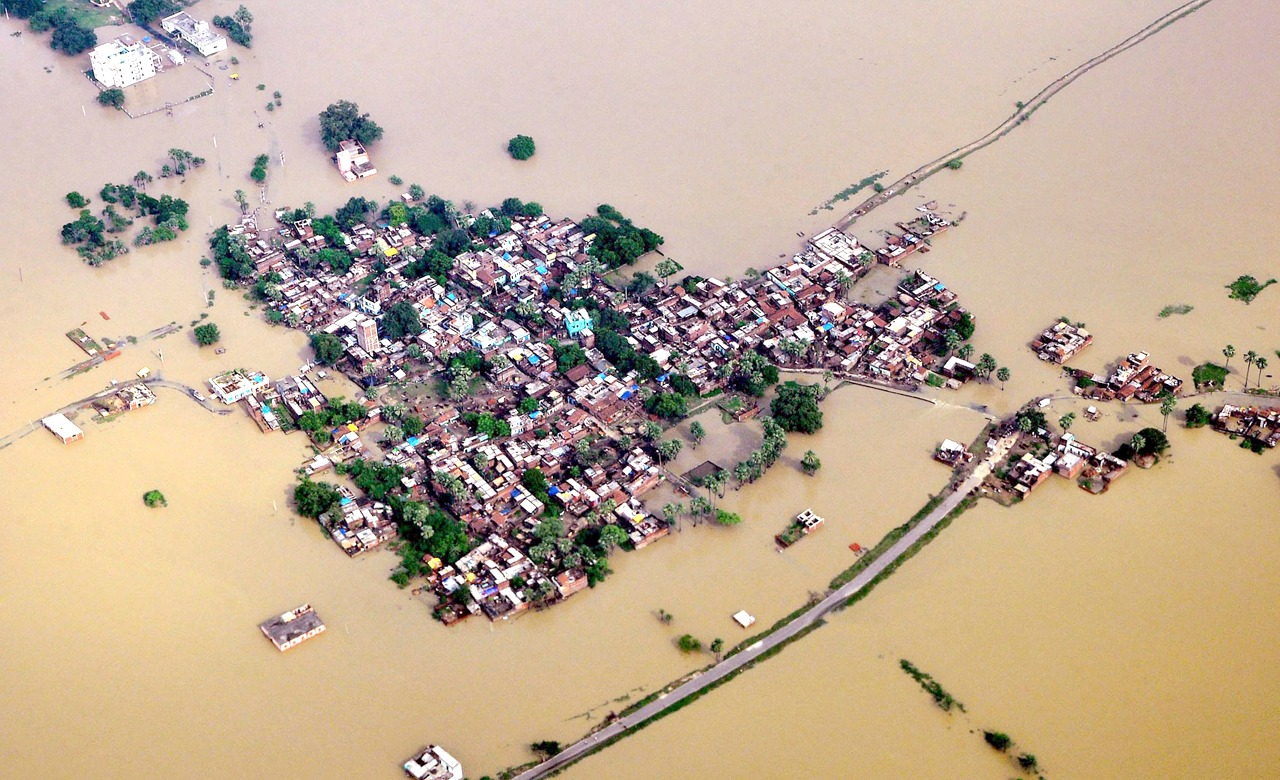
Spatial or geographic data can be gathered, analyzed and presented digitally using GIS technology where decision-makers may visualize, interpret and comprehend the relationships, patterns and trends…
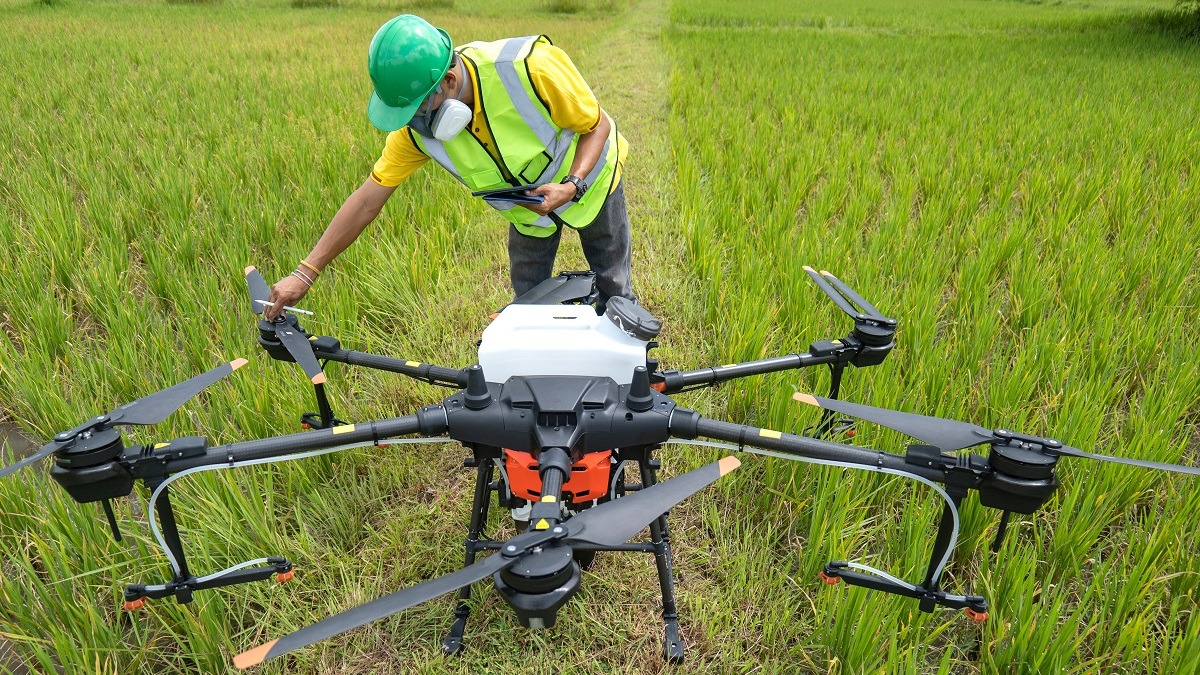
As the global population continues to grow, the agriculture industry faces the pressing challenge of meeting the rising demand for food while minimizing its environmental impact…
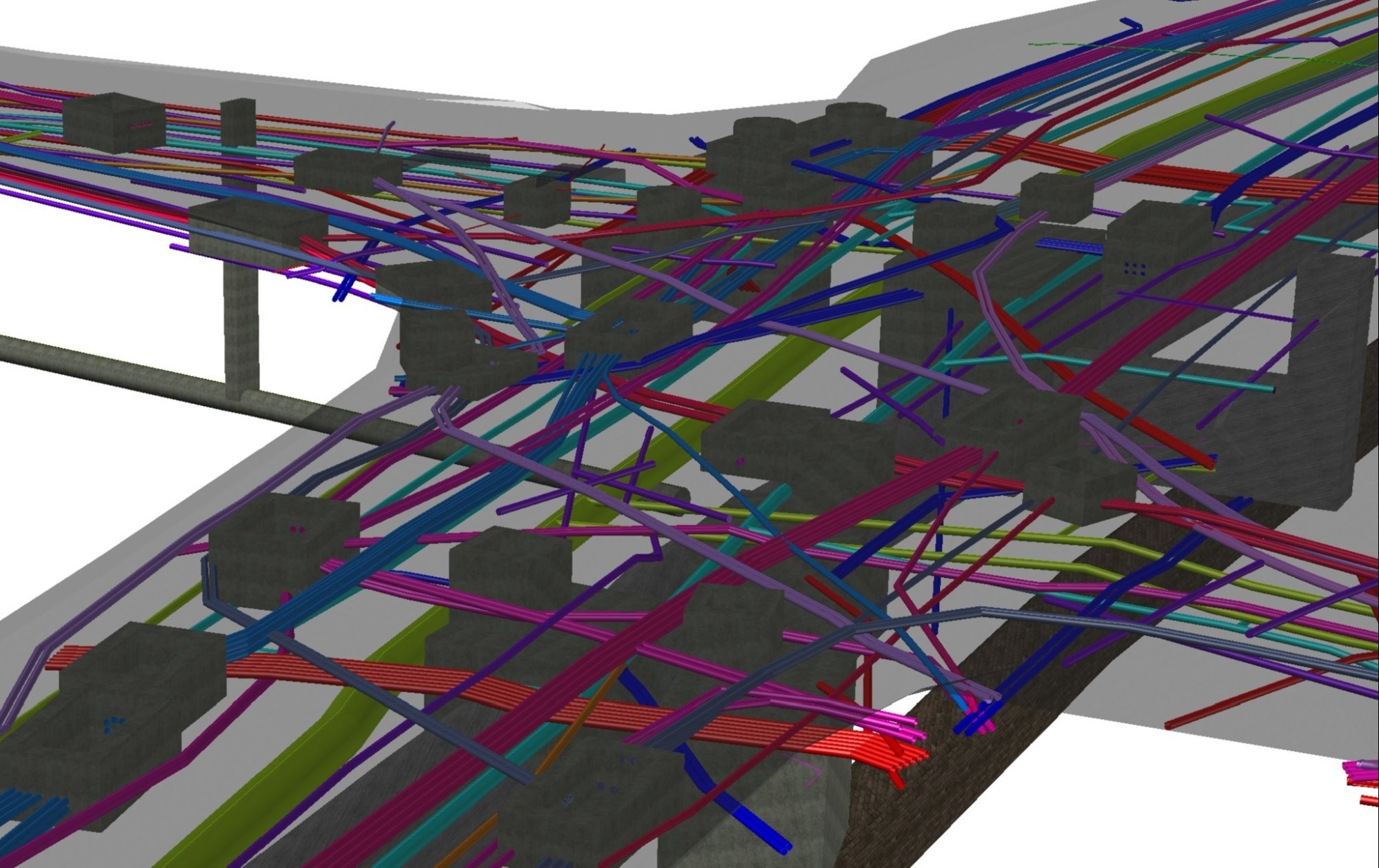
Utility mapping is the process of creating accurate, up-to-date and comprehensive maps of underground utilities and infrastructure where these utilities encompass a wide range of systems…
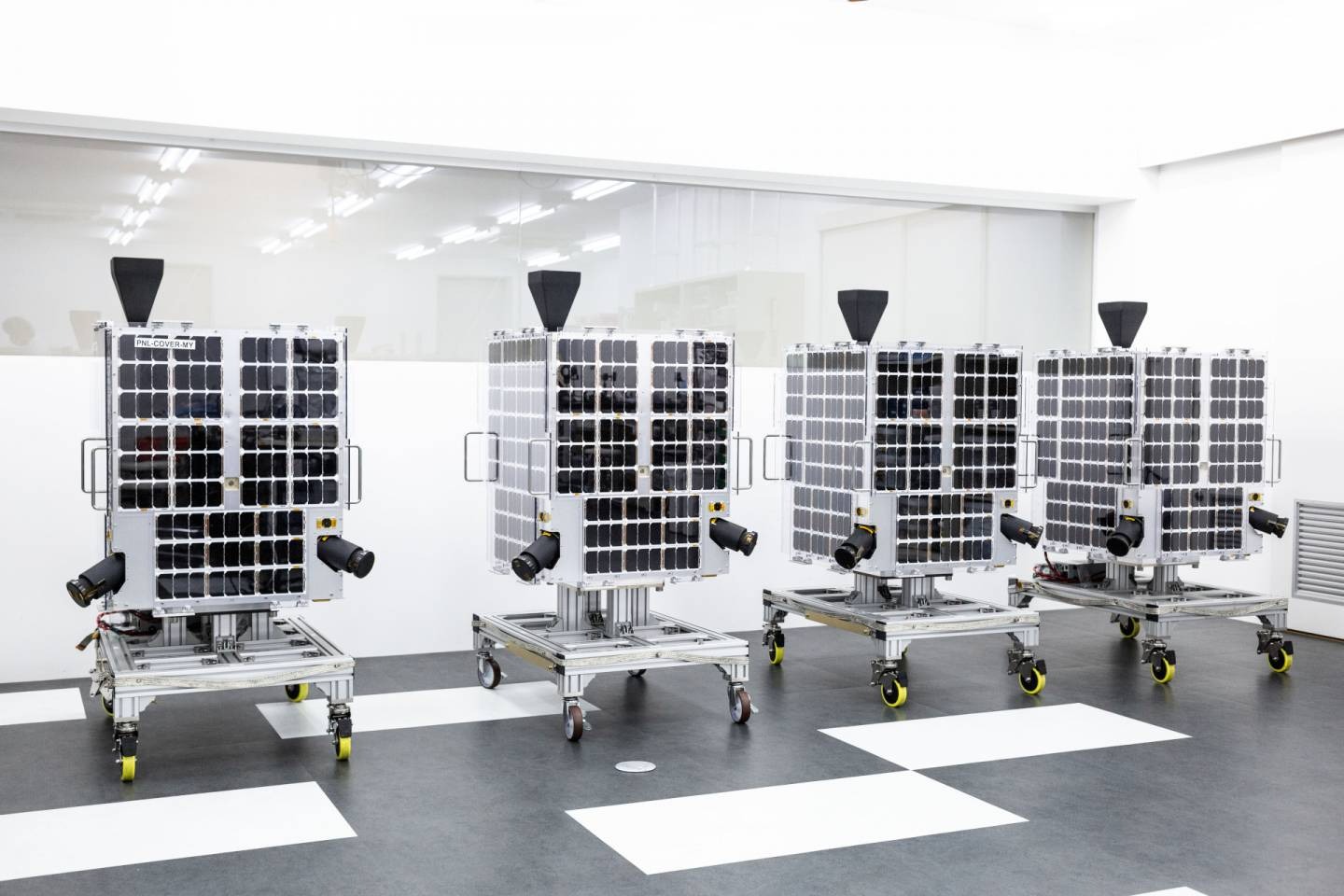
GRUS is a significant advancement in satellite technology created to satisfy the expanding needs of the GIS sector where the satellite which was created by top…
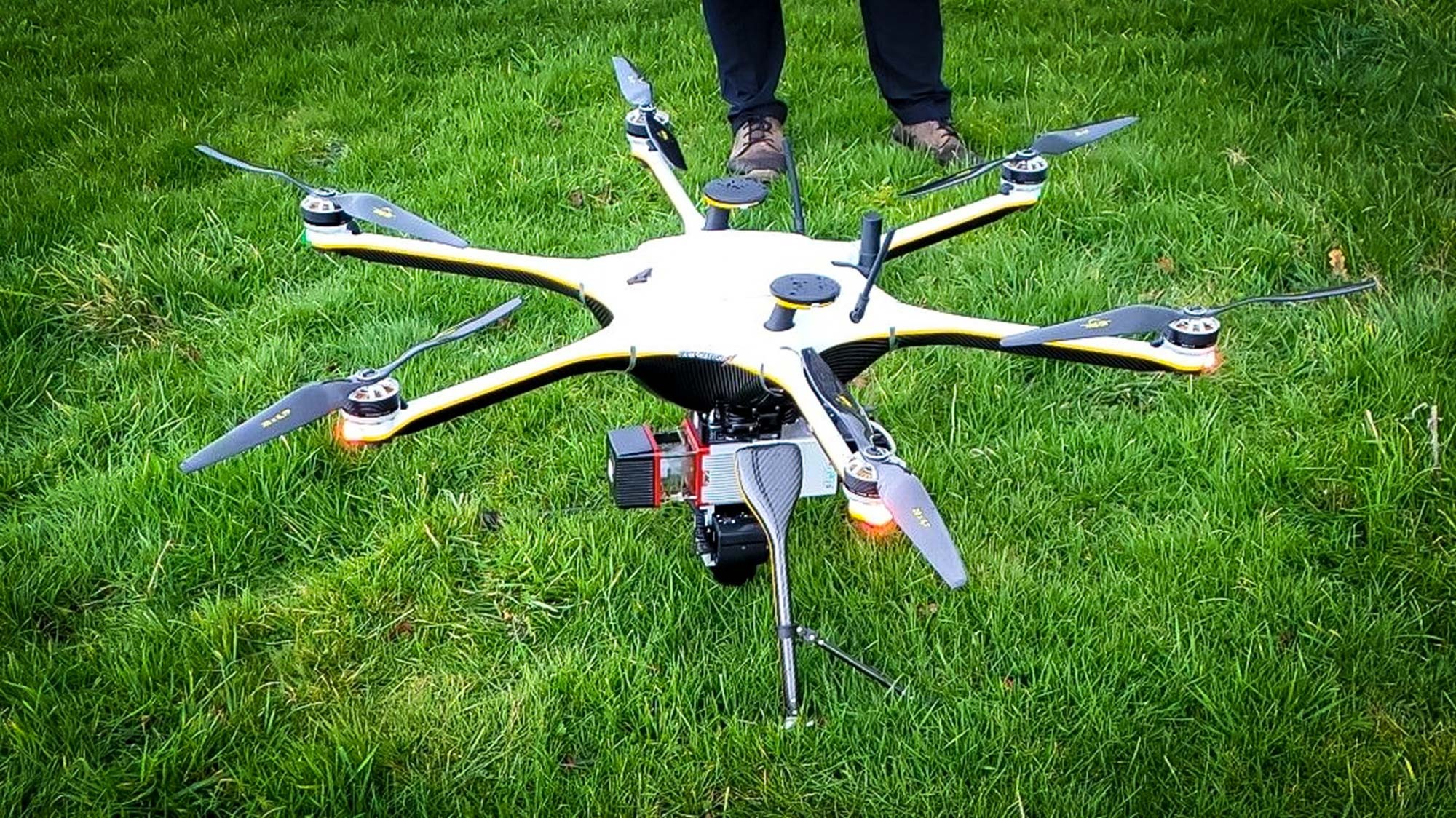
Drone surveys have been around since the early 20th century but they have come of age as a GIS powerhouse in the last 20 years. The…